Known Unknowns of Quantum Physics the Nobel Prize Committee Gets Wrong
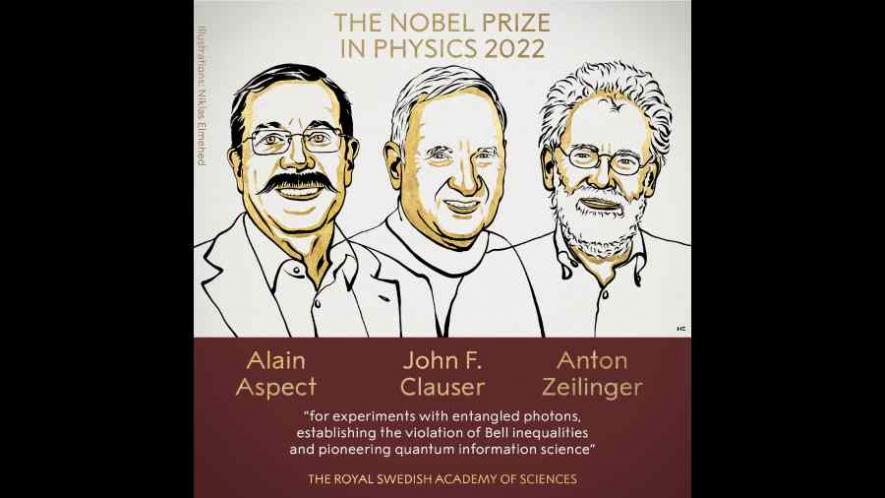
Image Courtesy: Twitter/@NobelPrize
The Nobel Prize in Physics this year was awarded to John Clauser, Alain Aspect and Anton Zeilinger “for experiments with entangled photons, establishing the violation of Bell inequalities and pioneering quantum information science”. Their experiments explored the foundations of quantum mechanics, the most precise theory of atoms and elementary particles known to humanity. Experimental techniques pioneered by them have led to an explosion of further experiments, and development of new technologies. The recognition for their achievements was long overdue. The official statement of the prize, and the publicity around it however misrecognise the significance of their work for further research in physics. The official statement makes a wrong claim about the so-called hidden variables theories. News reports about the prize misrepresent the importance of questions physicists like Albert Einstein and John Bell have raised about the dominant Copenhagen interpretation of quantum mechanics.
Known Unknowns of Quantum Mechanics
There are features of quantum mechanics which sharply distinguish it from other theories in physics. First, its formalism is more abstract. Other theories in physics represent relevant physical quantities directly in terms of real mathematical functions. The values of these quantities, and relationships among them, can be read directly from the formalism. This is not the case in quantum mechanics. The quantum wave function, which corresponds to the state of a quantum system, is mathematically a complex quantity, and has only an indirect physical interpretation.
As shown by David Bohm, the complex character of the wave function is necessary for its probability based interpretation in quantum formalism. The gap between the mathematical formalism of the theory and physical reality is filled by the Copenhagen interpretation elaborated by Niels Bohr, Werner Heisenberg, Max Born, Paul Dirac, etc. who can rightly be crowned among the founding fathers of quantum mechanics. The other group of founding fathers, notably, Einstein, deBroglie, Planck, and to some extent Schrödinger, raised serious doubts over the Copenhagen interpretation.
The other distinguishing feature of quantum mechanics is the central role of observation and measurement in it. Non-quantum theories of physics work with a realist understanding of measurement. Attributes of physical systems like energy, angular momentum, position or momentum have values, irrespective of whether a measurement is made or not. The measurement process discovers these pre-existing values. A consequence of this realist reading of measurement is that measurement processes and experimental observations are not considered integral to the theoretical understanding.
Experimental observations and measurements have a very different place in quantum physics. There are quantum states of a physical system for which an attribute has no particular value. The state is said to be containing a probability distribution that corresponds to all possible allowed values. For example, spin of an electron can have only two values, either up, or down. Nevertheless, an electron can exist in a state built up of both these values. When a measurement is made, only one of the values is found. Since before the measurement, the electron spin did not have this value, it is as if the measurement process ‘co-creates’ this value. This process of co-creation is inherently undeterministic and random. The same measuring apparatus, acting on the same state, returns randomly different values from among the allowed values.
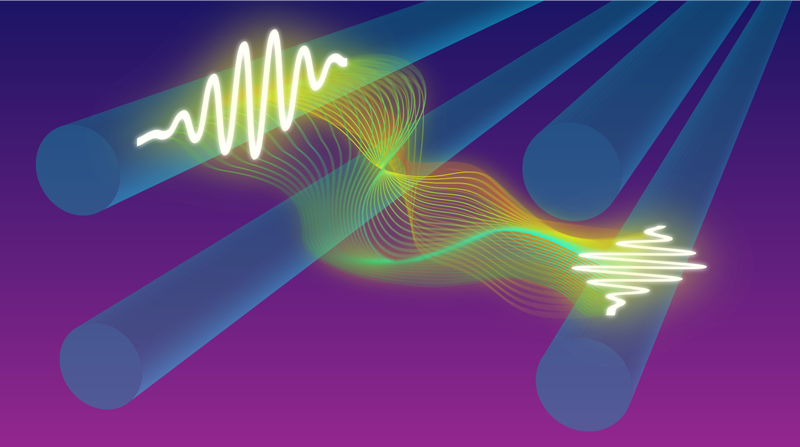
A visual representation of entangled photons travelling in different directions. Image courtesy physics.aps.org
However, if a second measurement immediately after the first measurement is made, the result is always the value found in the first measurement. Hence, the measurement process does act on the state of the system and changes it. It is loosely said that the measurement process ‘collapses’ the wave function, from a state corresponding to a probability distribution of all possible values of the attribute, to a state corresponding to a particular value. Before its collapse in a measurement, the wave function evolves deterministically according to Schrödinger equation. The collapse disrupts this smooth evolution, and plunges the system into a random process. Heisenberg’s uncertainty relations, which put a lower limit on how accurately certain pairs of quantities can be measured simultaneously, are a consequence of this randomness.
Another unique feature of quantum mechanics is entangled states which arise from two or more interacting particles, and in which the attributes of two particles are correlated and cannot be separated from each other. Clauser and Aspect’s experiments used entangled states of two photons (particles of light) which are emitted simultaneously in two opposite directions from certain kinds of excited atoms. Once established, their entangled character persists, even when the two travelling particles are far apart.
Entangled states challenge our normal understanding of a whole and its parts. Consider a gas enclosed in a cylinder. According to the kinetic theory of gases, molecules of the gas interact with each other through collisions. In the process, certain emergent properties of the gas, like its pressure and temperature arise, which are properties of the whole of the gas, rather than of its constituents. Molecules of the gas nevertheless retain their individual attributes like their energy and momentum. When a disturbance is created in one part of the gas, it travels through the gas according to well-known laws of physics applied locally at every point in the gas. Any such disturbance cannot travel faster than the speed of light in vacuum according to the theory of relativity. Both of these properties, retention of individual attributes of the constituents, and local character of any physical process through the whole, are absent in an entangled state.
The experiments of Clauser and Aspect have unambiguously established that when a measurement is made on either of the two well-separated particles of an entangled state, then the wave function of the entangled state collapses simultaneously all over, i.e. it is a non-local process.
It is obvious that there are gaps and mismatches in the quantum picture of reality. First is the deterministic evolution of the wave function according to Schrödinger equation, and its sudden ‘collapse’, leading to random outcomes during a measurement. Second is the non-local property of the wave function which contradicts the locality required by special relativity. The third one, not discussed here, is the lack of a precise understanding of the difference between microscopic systems described correctly by quantum mechanics, and large macroscopic systems which when described by quantum physics lead to absurdities like Schrödinger's cat, which is forced to be dead as well as alive when no one is observing it.
The EPR Paradox and its Fruits
The orthodox interpretation of quantum physics gets right the consequences of these known unknowns, but is otherwise silent about them. It does not provide even a language to raise pertinent questions about these phenomena. This silence has been tweaked open by the line of research which started with a paper in 1935 by Einstein, Podolsky and Rosen (EPR). The EPR argument was crucially transfigured theoretically by John Bell in 1964, so that it provided an empirical test on the foundations quantum mechanics.
Clauser and Aspect in the 1970s and 1980s experimentally explored the result discovered by Bell. Since experiments have confirmed predictions of quantum mechanics, it is generally claimed that doubts and concerns raised by Einstein and Bell were misplaced and have been laid to rest. This, however, misrepresents their concerns. They may have advocated specific conceptions which turned out to be incorrect, but their efforts clarified what is at stake.
EPR start with a quantum entangled state of two particles travelling in opposite directions. Momenta of the two particles are correlated in this state. For example, if one of them is found to be having momentum p, the other is bound to have momentum –p. This means that the measurement of the momentum of the first particle also provides the value of the momentum of the second particle without disturbing it in anyway. By this argument, EPR try to undercut the claim that a measurement necessarily disturbs the system. Their crucial assumption is locality, so that measurement on the first particle cannot have any impact on the second particle far away. They interpret the result of the indirect measurement on the second particle in a realist way, by claiming that since it is found without disturbing it in any way, it must have had the measured value before it was measured. Since the representation of an entangled state in quantum formalism provides no clue about this value, it must be incomplete.
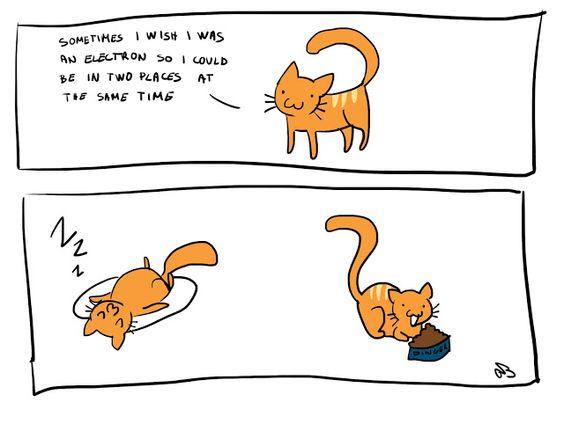
A Happy Version of Schrödinger's Cat. Image courtesy thefinchandpea
It should be noted that the EPR do not making any experimental claim different from quantum mechanics. So, there is no question of any experiment nullifying EPR. Their result is in the form of an interpretation, which holds true if the principle of locality is valid.
Bohr’s counter to the EPR argued that they were wrong in thinking that the wave function of the second particle was in some sense independent of the measurement on the first particle because the two were far away from each other. There exists only one wave function of the entangled state of both the particles. This wave function collapses and leads to correlated but random values when measurement is made on any one of the particles.
David Bohm in his textbook on quantum physics modified the EPR argument to an electron and a positron emitted from a decaying pion. In this case measurements are done on particle spins, which in principle are easy to find out than momenta or positions. John Bell allowed the spins of the two particles to be measured in arbitrarily different directions, and derived a remarkable result named after him. It turns out that all possible theories which satisfy the locality condition of special relativity and have additional ‘hidden variables’ satisfy a constraint, which is explicitly violated by the quantum mechanical prediction. Since experiments of Clauser and Aspect agree with this prediction, these rule out any local hidden variable theory. Their experiments also show that an entangled state and its collapse are indeed non-local phenomenon.
It needs to be noted that the most investigated hidden variable theory, the pilot wave theory of deBroglie and Bohm is an explicitly non-local theory. Experiments do not rule it out. The Noble prize press statement is wrong in claiming that ‘quantum mechanics cannot be replaced by a theory that uses hidden variables’. It is remarkable that even the statement by a physics institute like CERN fail to distinguish between local and non-local theories and misrepresent Bell’s theorem as valid for all hidden variable theories.
Boxing With Einstein’s Straw Man
Since Einstein is undoubtedly the most celebrated physicist of the twentieth century, it makes for good publicity to claim that he has been proved wrong. This year’s Nobel prize in physics too has led to such claims. The EPR tried to prove that quantum theory is incomplete on the assumption of locality. Experiments of Aspect and Clauser have shown that no local theory can describe entangled states. Hence, EPR’s assumption that the physics of the micro-world is local is wrong. On the other hand, while the quantum formalism correctly gets the non-local character of the micro world, it tells us nothing about its physics; what kind of physical state is it, what physical principles does it follow, etc. For all we know, some alternate theory may turn out to be right. So, in a way quantum physics remains incomplete, but not due to the reasons put forth by the EPR.
It is only after sustained questioning by scientists like Einstein and Bell that the non-local character of the quantum physics has come to the fore. The point perhaps is not between which group of scientists is right, and which one wrong. Such claims that imagine debates among scientists as winner takes all duels miss their significance.
The history of science shows that our understanding of physical reality has changed with time. The Newtonian mechanistic picture of quasi-rigid bodies, interacting with each other according to deterministic laws, was replaced by Maxwell-Einsteinian picture of fields and point particles, in the late nineteenth-early twentieth century. Quantum physics provides another very different picture of the micro-world. These changes have been revolutionary, but not random or arbitrary. Rather, they are a manifestation of deeper and wider spheres of human knowledge, as it has expanded to previously unknown domains.
Experimental techniques of Clauser, Aspect and Zeilinger have opened a new world of quantum technologies based upon entangled states. This in itself is a significant result of their work, and is rightly noted by the Nobel prize committee. However, there is another consequence of their work. Experiments with entangled states are also likely to help us understand what exactly these states are. And that knowledge is likely to be as revolutionary in its implications as were the transition from the Newtonian to Maxwell-Einsteinian picture of reality. The adventure of unpacking mysteries of the micro world is still on. Quantum mechanics may prove to be only a necessary and useful way station.
The author teaches physics at St Stephen’s College, University of Delhi. The views are personal.
Get the latest reports & analysis with people's perspective on Protests, movements & deep analytical videos, discussions of the current affairs in your Telegram app. Subscribe to NewsClick's Telegram channel & get Real-Time updates on stories, as they get published on our website.